During his undergraduate years, Rico Pohle studied experimental physics at the Dresden University of Technology in Germany. On taking his first courses in English there, he was introduced to the phenomena of magnetism and superconductivity.
“These subjects were very interesting and immediately aroused my curiosity because they sounded like science fiction or magic,” says Pohle. “I had no idea how they worked. And I wanted to understand the internal physical processes which led to their effects.”
But as his studies progressed, he realized experimental physics did not give him many opportunities to investigate what was happening inside the materials on which he was experimenting. Though he could measure a certain feature of a crystal, for instance, and get an accurate result, it did not necessarily reveal much about the crystal in a way that would help him grasp its underlying physics.
A break from physics
At the age of 14, Pohle had taken up karate and went onto become an avid practitioner. This sparked an interest in Japan in general and martial arts in particular, and so he availed himself of the opportunity to also study Japanese at university. When an occasion arose to visit Okinawa—the birthplace of karate—Pohle took a break from physics and went there for a year to practice karate and to study Japanese, as well as Japan’s history and culture.
During his time in Okinawa, he heard about the Okinawa Institute of Science and Technology (OIST), a new interdisciplinary graduate school that was preparing to open. “This was like a dream,” says Pohle. “I realized I could study physics, practice karate, and conduct research, which made it a perfect fit for me.”
He was especially impressed with the university’s aim to help Japan become more international in outlook through the encouragement of interdisciplinary collaboration, and by providing courses and teaching in English. “In fact, OIST has been designed and built to encourage such collaboration,” says Pohle. “Researchers at different labs are encouraged to talk about and discuss their work with colleagues from other labs. This helps create new associations and generate new ideas.”
After returning to Germany and completing his studies to obtain a Diploma (equivalent to a Master of Science in Physics), he applied to enter OIST to pursue a Ph.D. in Physics and was accepted.
OIST provided a series of lab rotations where Pohle could conduct research for three to four months in different labs to help him decide in which area of physics he wanted to specialize. During one such rotation, he joined a lab conducting theoretically studies in condensed matter physics.
Theoretical physics
Condensed matter physics is the field of physics that studies the macroscopic and microscopic properties of matter in solid and liquid forms. It uses the laws of quantum mechanics to predict the collective behavior of large numbers of interacting particles such as electrons, atoms, or molecules. And it explores how these interactions lead to various phenomena such as magnetism and superconductivity.
“I found this very interesting, and I could also use my programming skills to simulate physical systems using a computer,” Pohle explains. “This gave me the ability to ‘see’ and study physical concepts in a well-controlled way in the form of simulated experiments on a computer. I’m able to control variables like temperature, particle interactions, and degrees of freedom of electrons, for example, which are difficult to do in a physical experiment.”
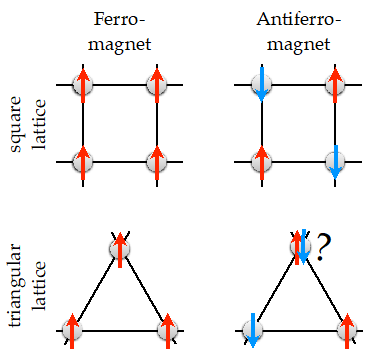
Fig.1 Comparison of spin configurations on square and triangular lattices. Ferromagnetic interactions align spins, while antiferromagnetic interactions arranges nearest-neighbor spins antiparallel. This induces frustration on the triangular lattice, as seen for spin (‘?’) which cannot decide whether to point up or down.
After OIST, he stayed in Japan to carry out post doctorial research at the University of Tokyo and Waseda University where he explored the quantum regime of many body physics, which involves how a large number of particles such as atoms or electrons behave when they interact with each other. There are different ways to study these problems, and at OIST he had learned classical and semi-classical methods. Now he could add a third major approach to tackle these puzzles. He also used these methods to study phenomena occurring in highly frustrated magnets (Fig. 1). These are magnets where conflicting interactions do not allow magnetic spins to easily align along the same directions—something that would come in useful later in his research on quantum spin liquids.
Quantum spin liquids
More recently, Pohle has become especially interested in quantum spin liquids (QSL), an exotic phase of matter which is likely to be found in the class of materials known as frustrated magnets, as was noted earlier (Fig. 1 above).
To explain QSL, he uses the analogy of the three phases of water: gas, liquid, and solid or ice (Fig. 2). In the case of gas, the water molecules are separated some distance from each other, so the interaction between them is weak. In the liquid phase, the molecules come closer together, but they do not order themselves into any specific form. Then in the solid phase where the molecules are ordered, you get an ice crystal.
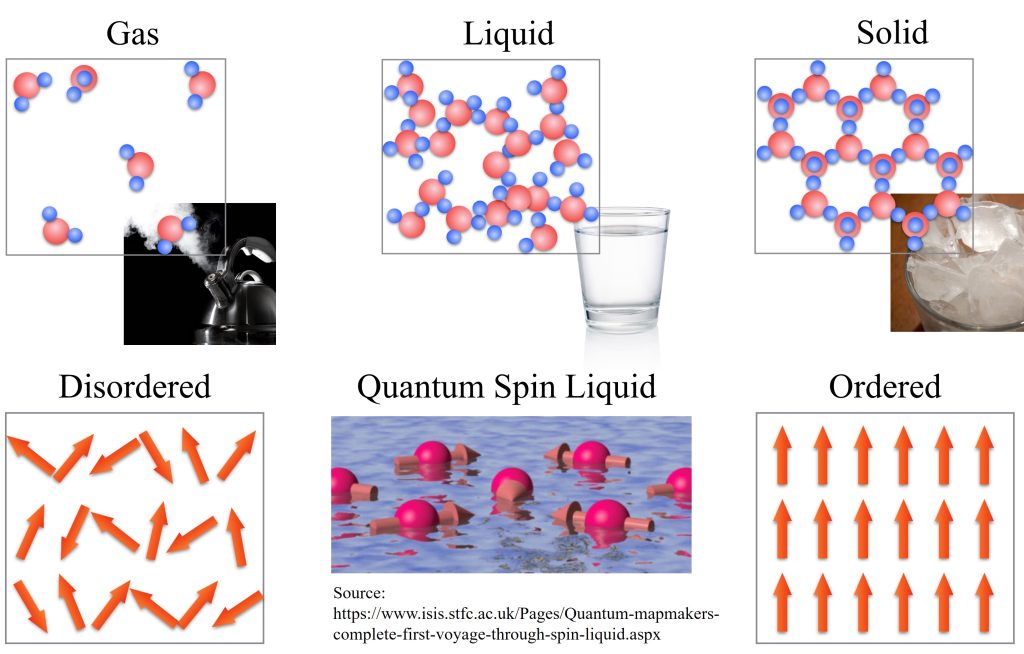
Fig. 2 Magnetic spins in condensed matter can exist in three phases (analogous to those in water) according to the strength of their interactions.
“In this phase, you also have various quantum effects emerging,” says Pohle. “That’s why we call it a quantum spin liquid.”
Entanglement
One especially curious effect is the phenomenon of long-range quantum entanglement. This is where magnetic spins remain strongly connected and influence each other even when far apart.
“Think of a crystal with a huge number of electrons that are all entangled,” says Pohle. “You change the spin of one electron and all the electrons in the crystal immediately change their spins. This is a state or property which occurs in a quantum spin liquid.”
Researchers are particularly interested in the phenomenon of entanglement because it could help, for example, in the development of fault-tolerant quantum computers, as the topological properties of the crystal make it resistant to certain types of noise and decoherence—two challenges the development of quantum computing still face.
Advanced research using Fugaku
Currently, Pohle works as a project Assistant Professor at Keio University where he is developing and using strong numerical methods to solve quantum many-body problems that involve significant entanglement.
The sub-group of researchers at Keio University to which Pohle belongs is part of a new program that began in spring 2023 called the Program for Promoting Research on the supercomputer Fugaku, Japan’s and one of the world’s fastest supercomputers. Nine co-implementing institutes, including Keio, under the directorship of the High Energy Accelerator Research Organization known as KEK, are taking part in the advanced project, and are conducting large-scale collaborative research ranging from subatomic particles and nuclei to condensed matter physics.
The program is one of an expanding number of research projects under the Joint Institute for Computational Fundamental Science (JICFuS). Although JICFuS has traditionally focused on physics research using supercomputers like Fugaku, this new program includes research using machine learning, and it is exploring synergies that may exist between supercomputers and quantum computing. Associate Professor Yusuke Nomura’s Group at Keio, where Pohle is conducting his research, is known for its use of machine learning techniques in the framework of materials science. This environment is enabling Pohle to explore and better understand exotic states of matter and their relevance to various materials.
Thinking of the Future
Looking ahead, Pohle notes that research into high-temperature superconductivity, for instance, has been going on since before he was born. The motivation behind this lies in the prospect that if the superconducting state could be realized at room temperature, it would change everyone’s lives by reducing the cost of generating, storing, transporting, and using electricity, and enabling, for example, new forms of transportation employing magnetic levitation.
“So a lot of research has and is still being carried out on this phenomenon to try and understand the physics behind it, yet it’s still not understood completely,” says Pohle. “If we are able to fully grasp the underlying mechanisms, then we could produce materials to make superconductivity practical.”
At the same time, and in one sense, at least, “Quantum spin liquids can be thought of as the little brother of superconductors, because they are somewhat related” says Pohle. Understanding quantum spin liquids, then, might help researchers understand superconductivity.
“In any case,” he adds, “I am interested in whatever happens along the way. I believe quantum spin liquids exhibit exotic physical properties that are at least as rich as those in superconductors. And I can imagine that in the future quantum spin liquids will also lead to new technological advancements as much as they are expected from superconductivity.”